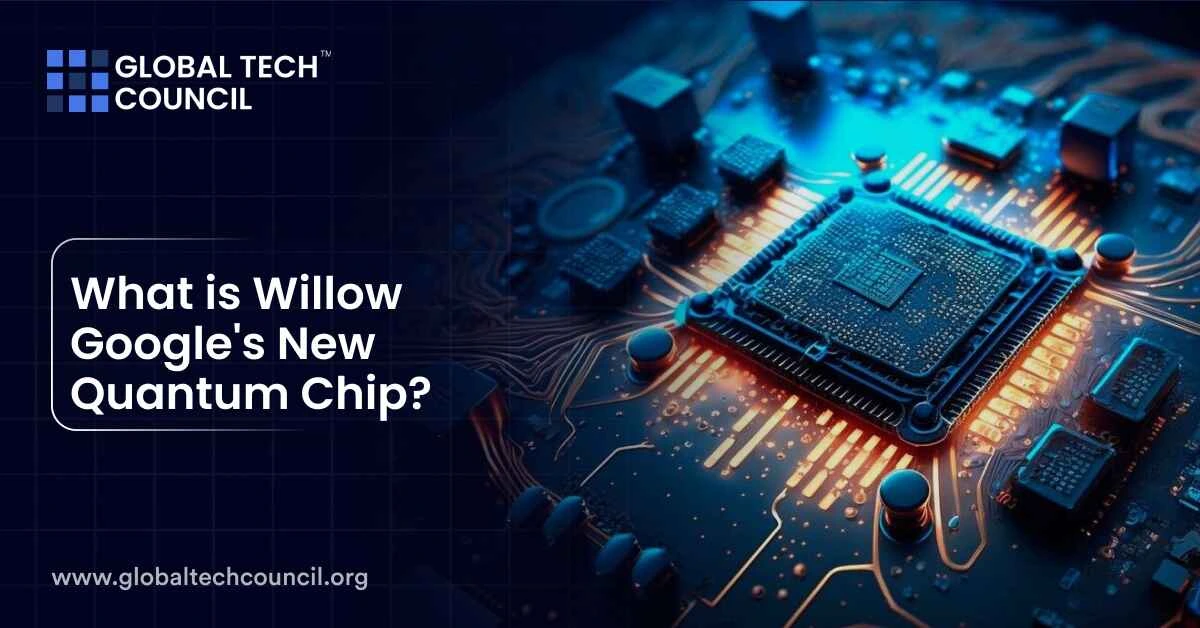
Google has introduced a groundbreaking quantum computing chip named Willow, which represents a massive leap in computational technology. Quantum computing has been one of the most challenging yet promising frontiers in modern science. Willow brings new possibilities by solving long-standing issues like error correction and scaling, and its performance has set new benchmarks in the field.
A Brief Introduction to Quantum Computing
Quantum computing relies on quantum mechanics to carry out calculations much faster than classical computers. Traditional computers use binary bits, represented as 0s and 1s. In contrast, quantum computers use qubits. Qubits have a special property called superposition, which lets them exist in multiple states at once. This ability enables quantum computers to handle massive amounts of data simultaneously, solving problems at an exponentially higher speed.
However, quantum computing has been hindered by two major challenges: errors and scalability. Qubits are extremely delicate and prone to environmental interference, which leads to errors during computation. Adding more qubits typically increases error rates, making it difficult to scale quantum processors. This is where Willow stands apart.
Introducing Willow: A Quantum Leap in Computing
Willow is Google’s latest quantum chip featuring 105 qubits. Unlike its predecessors, Willow not only increases the number of qubits but also drastically improves their reliability. The chip achieves an exponential reduction in error rates, a feat that has eluded researchers for decades. This advancement is critical for building larger and more practical quantum systems.
The 30-Year Challenge: Cracking the Error Code
Quantum computing promises revolutionary advancements, but it faces a significant roadblock: errors. These errors stem from the fragile nature of qubits, the building blocks of quantum computers. Unlike traditional computing bits that are stable and binary (either 0 or 1), qubits can exist in superpositions of states, such as 1, 0, or a mix of both. However, this power comes with a vulnerability. Qubits easily lose their quantum state when they interact with their environment, making large-scale, error-free quantum computing a daunting challenge.
Understanding the Error Problem
As more qubits are added to a system, they introduce more opportunities for error. Instead of improving performance, the system often becomes unstable and starts behaving like a classical computer. This scaling problem has limited the practical application of quantum computers for decades. To address this, researchers have focused on quantum error correction—a method to detect and fix errors before they disrupt computations. Despite years of effort, creating systems that improve performance as they scale remained out of reach until now.
This error poses a great threat to cybersecurity. To mitigate the risks, consider getting cybersecurity certifications from renowned organizations like the Global Tech Council.
Willow’s Breakthrough in Error Suppression
Google’s Willow chip is a quantum computing breakthrough that addresses the scaling challenge head-on. By leveraging advanced quantum error correction, Willow reduces errors exponentially as the number of qubits increases. The team achieved this by arranging qubits into grids of increasing size: 3×3, 5×5, and 7×7. Each step saw the error rate cut in half, an achievement researchers have pursued for nearly 30 years.
The secret lies in Willow’s combination of innovative hardware and cutting-edge software. Its error correction algorithms, powered by machine learning, analyze errors in real-time and correct them faster than they can accumulate. This real-time correction is crucial because, without it, errors would multiply and disrupt computations.
The Significance of “Below Threshold”
Willow’s success led to a landmark achievement in the quantum computing field: operating “below threshold.” This milestone, introduced by theorists in the 1990s, refers to the point where error correction begins to outpace error generation. In other words, instead of errors increasing with system size, they decrease, allowing the system to improve as it grows. Achieving this threshold has been an elusive goal in quantum computing until now.
Google demonstrated this by showing that Willow’s arrays of qubits—when error-corrected—last longer and perform better than individual qubits. For instance, the 7×7 lattice on Willow doubles the lifetime of its best physical qubit, a clear sign of successful error correction. This improvement transforms the limitations of previous systems into a scalable advantage.
Why This Matters for the Future
Operating below the error threshold is a pivotal step toward building practical, large-scale quantum computers. It means researchers can now confidently scale up quantum systems, knowing that performance will improve with size. This progress not only validates decades of theoretical work but also lays the groundwork for tackling real-world problems in areas like drug discovery, material science, and artificial intelligence.
Willow’s advancements represent more than just a milestone—they signify a shift in how we approach quantum computing. For the first time, the field has proof that scaling up can lead to better performance rather than more challenges. This achievement sets the stage for quantum computers to eventually surpass their classical counterparts in solving complex, meaningful problems.
How Fast Is Fast? Willow’s Record-Breaking Feat
Google’s Willow quantum processor has shattered records and set new benchmarks in quantum computing. One of its most striking achievements is its performance in Random Circuit Sampling (RCS)—a test specifically designed to highlight the computational advantages of quantum systems over classical computers. Willow’s success in this area not only showcases its raw processing power but also provides a glimpse into the transformative potential of quantum technology.
Willow’s Performance in Random Circuit Sampling (RCS)
Random Circuit Sampling is a critical benchmark used by quantum computing researchers. It involves generating and processing highly complex quantum circuits with random inputs. These computations are extremely challenging for classical computers because they require exponential scaling of resources as complexity increases. RCS serves as a litmus test for whether a quantum computer is performing genuinely quantum tasks that classical systems cannot replicate.
In this benchmark, Willow demonstrated a historic achievement. It solved the computation in under five minutes, a task that would take one of the fastest classical supercomputers, such as Frontier, approximately 10 septillion years. To break that down, 10 septillion is a 1 followed by 25 zeros—10,000,000,000,000,000,000,000,000 years. This time frame vastly exceeds the age of the universe, which is about 13.8 billion years.
The comparison underscores Willow’s dominance in computational power. Even with highly favorable assumptions for classical supercomputers—such as unlimited memory, ideal storage, and optimal parallelization—Google estimates that it would still take over a billion years to achieve the same result.
5 Minutes vs. 10 Septillion Years: What Makes Willow So Fast?
Willow’s speed stems from its ability to harness quantum mechanics to perform computations in parallel, something classical systems cannot do efficiently. Classical computers process bits in sequential states of 0 or 1. In contrast, qubits—used in quantum computers—can exist in superpositions of multiple states simultaneously. This property enables quantum computers to explore a vast number of possibilities at once.
Several key innovations in Willow’s design amplify this advantage:
- Advanced Qubit Architecture: Willow features 105 high-quality qubits that operate with exceptional coherence times, meaning they can maintain their quantum states longer. This allows the system to perform more computations before errors occur.
- Optimized Connectivity: Each qubit in Willow interacts with others in a highly optimized manner, enabling efficient communication and reducing bottlenecks in calculations.
- Error Correction Breakthroughs: Willow incorporates state-of-the-art quantum error correction. This system detects and corrects errors in real-time, allowing computations to continue without disruptions. The exponential reduction in error rates as more qubits are added—a milestone known as “below threshold”—plays a crucial role in achieving this speed.
- Purpose-Built Fabrication: Willow was developed in Google’s specialized fabrication facility in Santa Barbara. This state-of-the-art lab focuses exclusively on building and refining quantum processors. Every aspect of the chip’s design, from its physical structure to the software that powers it, has been fine-tuned for maximum performance.
What This Speed Means for Quantum Computing
Willow’s record-breaking performance is more than a technical achievement—it has profound implications for the future of quantum computing. First, it solidifies the notion that quantum processors can outperform classical systems in specific tasks, a concept known as “quantum supremacy.” This milestone confirms that quantum computing is no longer limited to theoretical potential; it is now delivering tangible results.
The success of Willow also provides a glimpse into the practical applications of quantum computing. While RCS itself does not have direct real-world uses, it serves as a benchmark for more complex problems. The same principles that allow Willow to excel at RCS could be applied to fields such as drug discovery, material science, and optimization problems. For instance, quantum computers could help design more efficient batteries or simulate quantum systems to discover new medicines.
Additionally, this achievement has fueled competition among tech giants, including Microsoft, IBM, and Amazon, all of whom are working on their own quantum computing systems. However, Willow’s ability to consistently push the boundaries of what is possible sets it apart as a leader in the field.
Built for the Future: Behind Willow’s Development
Google’s Quantum AI Lab began in 2012 with a bold vision: to harness quantum mechanics and build a quantum computer capable of solving problems beyond the reach of classical systems. This journey required sustained innovation and collaboration, spanning over a decade. The team aimed to develop technology that could benefit society by advancing science, addressing real-world challenges, and powering transformative applications in fields like drug discovery, clean energy, and artificial intelligence.
Over the years, Google’s researchers laid a roadmap for progress, setting ambitious milestones. These included achieving “quantum supremacy” in 2019, where their Sycamore processor performed a computation faster than any classical computer could manage. While this marked significant progress, the team knew they had to address larger challenges—particularly the issue of scaling up qubits while reducing errors. This dedication ultimately led to the creation of Willow, a state-of-the-art quantum processor that represents a leap forward in the field.
The State-of-the-Art Facility in Santa Barbara
Willow owes much of its success to Google’s advanced fabrication facility in Santa Barbara, California. Inaugurated in 2021, this facility was specifically built to support quantum computing research and development. It is one of the few facilities globally that is entirely dedicated to manufacturing quantum chips from the ground up.
This lab provides an ideal environment for designing, fabricating, and testing quantum processors. By housing all processes under one roof, the team can quickly translate new ideas into physical prototypes. For example, engineers can create and test chips in cryostats—devices that keep them at near-zero temperatures—essential for quantum operations. This setup significantly accelerates the pace of experimentation and learning, giving researchers the tools to refine designs and iterate rapidly.
The Santa Barbara facility also focuses on collaboration between hardware and software teams. This integration ensures that every improvement in hardware directly supports advances in error correction and computation. By having these teams work closely, Google can optimize every aspect of its quantum systems.
The Role of System Engineering in Quantum Chips
Designing a quantum chip like Willow involves more than just increasing the number of qubits. Every component, from gates to qubit resets and readouts, must work seamlessly together. System engineering plays a critical role in ensuring that these elements perform reliably and integrate smoothly.
Engineers must optimize the architecture, balancing performance and scalability. For Willow, this meant addressing issues like qubit coherence times, which measure how long a qubit can retain its state. By improving the lifetimes of individual qubits from 20 microseconds in earlier chips to nearly 100 microseconds in Willow, Google has created a system that supports more reliable computations.
Moreover, the team prioritized quality over quantity. Rather than adding hundreds of low-quality qubits, they focused on building a system with 105 high-performing qubits. This approach ensured that Willow excelled in key benchmarks like quantum error correction and random circuit sampling. The integration of machine learning algorithms for error detection further enhanced system performance.
Quality Over Quantity: Why Reliable Qubits Matter
Why High-Quality Qubits are Essential
In quantum computing, simply increasing the number of qubits does not guarantee better performance. Unlike classical computing, where more cores or memory can directly improve speed and capability, quantum systems are far more sensitive. Qubits, the basic units of quantum computation, must work together in perfect harmony. Any instability in one qubit can introduce errors, which can then cascade through the system and undermine the entire computation.
For this reason, building reliable and high-performing qubits is more important than just focusing on quantity. Google’s Willow chip exemplifies this approach by prioritizing quality over numbers. Instead of aiming to create hundreds or thousands of qubits, Willow uses 105 qubits, each engineered to perform at a best-in-class level. This strategic focus ensures that the system delivers better overall results in tasks like error correction and benchmarking.
Willow’s Performance Benchmarks and Specifications
The Willow quantum processor achieves impressive benchmarks, demonstrating its superiority over earlier chips. A key indicator of its progress is the error reduction rate. Willow’s qubits are optimized to achieve exponential error suppression as the system scales up, a critical milestone in quantum computing.
As mentioned earlier, this chip uses advanced surface code technology, grouping qubits into grids like 3×3, 5×5, and 7×7 arrays. As these grids increase in size, Willow reduces the error rate by half with each step. This exponential improvement highlights the chip’s ability to overcome challenges that have hindered quantum computing for decades.
Key technical specifications of Willow include:
- Number of Qubits: 105, chosen for their reliability and high coherence times.
- T1 Times (Qubit Lifetimes): Nearly 100 microseconds, representing a fivefold improvement over previous-generation chips like Sycamore.
- Average Connectivity: 3.47, enabling efficient qubit interactions for complex computations.
These metrics indicate that Willow doesn’t just add more qubits to the system—it makes each qubit count.
How Willow Outperforms Previous Chips
Willow delivers significant improvements over Google’s earlier quantum processors. For instance, compared to Sycamore, Willow’s qubits are five times better in terms of coherence times. Coherence time measures how long a qubit can retain its quantum state before interacting with its environment and losing information. With nearly 100 microseconds of coherence, Willow sets a new standard for stability and reliability.
Moreover, Willow incorporates advancements in fabrication, calibration, and design. Google fabricated the chip in its state-of-the-art facility in Santa Barbara, which was purpose-built to optimize quantum chip development. Engineers focused on creating qubits that not only function well individually but also perform seamlessly within the larger system. This holistic approach ensures that the chip achieves maximum efficiency during real-time operations.
Willow also benefits from advanced error correction algorithms powered by machine learning. These algorithms continuously monitor qubits, detect issues, and correct errors before they escalate. As a result, Willow’s logical qubits (which combine multiple physical qubits) perform better than any individual qubit, doubling their effective lifespan.
Beyond the Hype: Real Challenges Still Ahead
What Willow Can’t Do Yet
Despite these achievements, Willow remains an experimental device. It has not yet performed any real-world, commercially relevant tasks. While benchmarks like RCS highlight its raw computational power, they do not translate directly to practical applications. Problems like drug discovery, material design, and optimization—areas where quantum computing is expected to shine—are still out of reach.
Willow’s advancements are groundbreaking, but the system operates in controlled environments that are optimized for specific tasks. Real-world applications will require quantum computers to function in less controlled scenarios and handle a broader range of problems. Achieving this goal will take years of further development and refinement.
The Gap Between Experimental Achievements and Practical Applications
The journey from experimental success to practical utility in quantum computing is long and complex. Willow’s achievements mark a milestone, but there is still a significant gap between these demonstrations and solving real-world challenges.
For instance, while Willow’s exponential error reduction is impressive, practical quantum computers need error rates to drop far lower than they currently are. To run large-scale, commercially useful algorithms, quantum computers must handle billions or trillions of operations without failure. Willow, like all current quantum systems, still falls short of this standard.
Moreover, most of Willow’s achievements rely on highly specific setups tailored for benchmarks. Real-world applications often involve less predictable conditions and require quantum computers to solve diverse and dynamic problems. Bridging this gap will involve not only improving hardware but also developing algorithms that can fully leverage quantum capabilities.
Error Rates Still Need Improvement
Error correction remains one of the biggest challenges in quantum computing. Willow demonstrates significant progress, cutting error rates in half with each increase in qubit grid size. However, today’s quantum systems still experience at least one error in every thousand operations. For practical applications, error rates must drop to one in a billion or even one in a trillion operations.
While Willow’s logical qubits already outperform individual qubits in terms of reliability, this is only the beginning. Scaling up quantum systems introduces new challenges, such as managing interactions between thousands or millions of qubits. Even small inefficiencies can compound as systems grow, making error rates a critical area of focus.
Google has acknowledged these limitations and continues to invest in solutions. The team uses machine learning algorithms to enhance error correction and has developed new fabrication techniques to improve qubit quality. However, achieving the necessary error rates will take years of sustained effort and innovation.
The Race to Real-World Impact: What’s Next?
Achieving “Useful, Beyond-Classical” Computations
Google’s ultimate goal for its quantum computing initiative is to achieve “useful, beyond-classical” computations. This refers to solving real-world problems that are impossible or impractical for classical computers to tackle. While Google has demonstrated the sheer computational power of its quantum systems with benchmarks like Random Circuit Sampling (RCS), the focus now shifts toward solving problems that have tangible value for society.
Until now, many quantum experiments have been purely theoretical or designed to test specific capabilities. For example, RCS demonstrates that Willow can vastly outperform traditional supercomputers on highly complex computations. However, these computations do not yet translate into real-world applications. Google’s next step is to combine this raw power with practical utility, creating solutions for industries ranging from healthcare to clean energy.
Real-World Applications of Quantum Computing
Quantum computing holds the promise of solving problems that are far too complex for classical systems. Google envisions applications in several key fields, where quantum systems could unlock breakthroughs that would otherwise take decades or remain impossible.
- Medicine and Drug Discovery: Quantum computers can simulate molecular interactions at a level of detail that classical computers cannot match. This capability could revolutionize drug discovery by allowing scientists to design new medicines more efficiently. For instance, researchers could model how specific molecules interact with proteins in the human body, speeding up the development of treatments for diseases like cancer or Alzheimer’s.
- Battery Design and Clean Energy: Developing more efficient batteries for electric vehicles and renewable energy storage is another promising application. Quantum systems can analyze the behavior of materials at the atomic level, identifying compounds that could store energy more effectively. These insights could lead to breakthroughs in battery technology, making renewable energy more accessible and sustainable.
- Fusion Energy: Quantum computers could also play a crucial role in advancing nuclear fusion, a clean and nearly limitless energy source. By simulating the behavior of plasma and magnetic fields in fusion reactors, quantum systems could help optimize reactor designs and accelerate progress toward practical fusion energy.
- Artificial Intelligence: Quantum computing can enhance AI in several ways. For example, it can speed up the training of machine learning models by solving complex optimization problems faster. Additionally, quantum systems can analyze large datasets more efficiently, helping AI systems make better predictions and decisions. This capability could benefit industries like finance, healthcare, and autonomous systems.
Examples of Problems Waiting for Quantum Solutions
The possibilities for quantum computing go far beyond these examples, influencing various aspects of human life and industry. Here are some specific challenges that could gain from quantum solutions:
- Global Logistics Optimization: Quantum systems could optimize supply chains and transportation networks, reducing costs and improving efficiency.
- Climate Modeling: Quantum simulations could provide deeper insights into climate change by analyzing complex interactions within Earth’s systems.
- Material Science: Scientists can use quantum systems to develop innovative materials with extraordinary characteristics, such as superconductors or ultra-light, highly durable materials for use in construction and aerospace.
- Encryption and Cybersecurity: Quantum computers could break traditional encryption methods, but they could also help develop quantum-proof cryptographic techniques to secure sensitive information.
Addressing Skepticism in the Quantum Field
While Willow’s achievements are remarkable, some experts caution against overhyping its capabilities. Critics argue that benchmark tests like RCS are tailored to highlight quantum advantages and may not reflect general performance. Additionally, Willow’s error rates, though improved, still need further reduction for practical applications.
Complementary, Not Replacement Technology
It’s essential to note that quantum computers are not meant to replace classical systems. Instead, they excel at specific tasks, such as optimization problems and quantum simulations, where classical computers struggle. The two technologies will work together to address complex challenges.
The Road Ahead for Google and Quantum Computing
Willow is a milestone, but it is not the final destination. Google’s Quantum AI team is already working on the next generation of chips, aiming for larger, more robust quantum systems. The company has outlined a roadmap that includes achieving commercially relevant quantum applications and building large-scale, error-corrected quantum computers.
To accelerate progress, Google is inviting researchers and developers to contribute through open-source tools and educational resources. Collaborative efforts will be crucial in overcoming remaining technical hurdles.
Conclusion
Willow represents a significant step forward in quantum computing. By reducing errors while scaling up qubits, it addresses some of the field’s most pressing challenges. Its astonishing performance in benchmark tests and improved reliability signal a promising future for quantum technology. While practical applications are still on the horizon, Willow brings us closer to unlocking the full potential of quantum computing, which could revolutionize medicine, energy, AI, and beyond. This journey is just beginning, and Willow is a testament to the incredible progress being made in this exciting field.